Developing & Manufacturing Oligonucleotides: An Overview
By Jack (Jie) Huang MD, Ph.D., CSTEAM Biotechnology
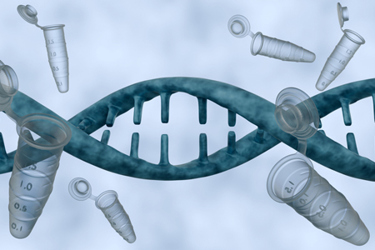
Oligonucleotides are short nucleic acid sequences, typically 20 to 100 nucleotides in length, synthesized for a variety of applications including diagnostics, therapeutics, and research.1 The development and manufacture of oligonucleotides requires rigorous methods to ensure their efficacy, safety, and quality.2 These molecules are essential tools in fields as diverse as gene therapy, molecular diagnostics, and synthetic biology. Ensuring high-quality production of oligonucleotides requires careful consideration of the sequence design, synthesis, purification, and quality control processes. Factors such as base composition, length, and potential secondary structure must be considered during the design phase to optimize performance3 Synthesis is typically performed using automated solid-phase techniques, followed by purification methods such as HPLC and PAGE to achieve high purity.4 Rigorous quality controls, including mass spectrometry and UV spectroscopy, are essential to confirm the integrity and functionality of oligonucleotides.5
Let’s take a closer look at key considerations to keep in mind.
Design And Development Of Oligonucleotides
Sequence Design
The design phase is important to ensure that the oligonucleotide meets the specifications required for its intended application. The following considerations are critical:6
- The oligonucleotide sequence must be complementary to the target sequence, whether it is DNA, RNA, or other biomolecules.
- The melting temperature (Tm) is calculated to ensure optimal hybridization. This is particularly important for therapeutic oligonucleotides, where stability and binding affinity are critical.
- Design sequences to minimize the potential for the formation of hairpins, dimers, or other secondary structures that may interfere with function.
- Consider incorporating modifications, such as phosphorothioate backbones or 2'-O-methyl groups, to enhance stability and resistance to nucleases, especially for therapeutic applications.
Preclinical Development
Preclinical studies are essential to assess the safety and efficacy of oligonucleotides prior to clinical trials. Key steps include:7
- In vitro studies: Assess the stability, binding affinity, and specificity of oligonucleotides in cell culture.
- In vivo studies: Animal studies are performed to assess pharmacokinetics (PK), pharmacodynamics (PD), and toxicity. Particular attention should be paid to the distribution, metabolism, and excretion (DME) of oligonucleotides.
Synthesis Of Oligonucleotides
Solid Phase Synthesis
The most common method for oligonucleotide synthesis is solid phase synthesis, typically using the phosphoramidite method. This involves:8
- The first nucleotide is attached to a solid support via its 3'-hydroxyl group.
- Successive nucleotides are added stepwise, and the 5'-hydroxyl group of the growing chain is activated and coupled to the 3'-phosphoramidite group of the incoming nucleotide.
- Phosphites are oxidized to stable phosphotriesters, and any unreacted hydroxyl groups are capped to prevent truncation of the product.
- Once synthesis is complete, the oligonucleotide is cleaved from the solid support and deprotected to remove any protecting groups.
Purification
Purification is essential to remove impurities such as truncated sequences and incomplete synthesis products. Techniques include:9
- Reversed-phase high performance liquid chromatography (RP-HPLC): separates oligonucleotides based on hydrophobicity, thereby isolating full-length sequences.
- Ion exchange chromatography: separates oligonucleotides based on charge differences, which are usually due to the length of the sequence.
- Polyacrylamide gel electrophoresis (PAGE): provides high-resolution separation of oligonucleotides and is particularly useful for therapeutic applications that require high purity.
Quality Control And Characterization
Analytical Methods
Quality control (QC) is a critical component of oligonucleotide manufacturing to ensure that the final product meets the required specifications. The following analytical methods are commonly used:10
- Mass spectrometry: confirms molecular weight and identifies any synthetic errors or modifications.
- UV spectrophotometry: measures the concentration and purity of oligonucleotides by assessing absorbance at 260 nm.
- Capillary electrophoresis (CE): provides high-resolution analysis of oligonucleotide purity and length.
- Nuclear magnetic resonance (NMR): provides detailed structural information and is particularly useful for modified oligonucleotides.
Stability Testing
Stability studies are essential to determine the shelf life and appropriate storage conditions of oligonucleotides. These studies should evaluate:11
- Temperature stability: Evaluate the stability of oligonucleotides at different temperatures, typically room temperature, refrigerated, and frozen conditions.
- Photosensitivity: Evaluate the effects of light exposure on the integrity of oligonucleotides.
- Long-term storage: Long-term stability studies are performed to determine the shelf life and optimal storage conditions.
Regulatory Considerations
Good Manufacturing Practices (GMP)
For oligonucleotides intended for therapeutic use, good manufacturing practices (GMP) must be followed. Key GMP considerations include:12
- Records: Maintain detailed records of all processes, from raw material procurement to final product testing.
- Validation: Ensure that all equipment, processes, and analytical methods are validated according to regulatory standards.
- Quality assurance (QA): Implement a strong QA system to oversee all aspects of production and ensure compliance with regulatory requirements.
Regulatory Submissions
Regulatory submissions must include comprehensive data on the development, synthesis, and testing of the oligonucleotide. Key components include:13
- IND application: Required prior to initiating clinical trials, the IND must include data on preclinical testing, manufacturing processes, and proposed clinical protocols.
- NDA: For therapeutic oligonucleotides, the NDA must include detailed information on clinical trial results, manufacturing processes, and quality control measures.
Advances And Future Directions
Novel Synthesis Technologies
Recent advances in synthesis technologies aim to improve the efficiency and scalability of oligonucleotide production. These synthesis technologies include:14
- Enzymatic synthesis: This offers a more environmentally friendly alternative to chemical synthesis, reducing the need for organic solvents.
- Microfluidic synthesis: This allows for the synthesis of multiple oligonucleotides in parallel, increasing yields and reducing costs.
- Automated platforms: Fully automated synthesis platforms are being developed to streamline the production process from design to final product.
Delivery Systems
Effective delivery of therapeutic oligonucleotides remains a major challenge. Advances in delivery systems include:15
- Lipid nanoparticles (LNPs): Widely used for siRNA delivery, LNPs protect oligonucleotides from degradation and enhance cellular uptake.
- Conjugates: Attaching oligonucleotides to targeting ligands such as peptides or antibodies can improve tissue specificity and reduce off-target effects.
- Polymer carriers: Biodegradable polymers offer a versatile platform for oligonucleotide delivery with the potential for controlled release and reduced toxicity.
Emerging Applications
The expanding use of oligonucleotides in gene therapy, personalized medicine, and synthetic biology highlights the need for continued innovation. Emerging application areas include:16
- CRISPR-Cas9 gene editing: Oligonucleotides are used as guide RNAs to direct Cas9 to specific genomic sites, enabling precise gene editing.
- mRNA therapeutics: Synthetic oligonucleotides are used to stabilize mRNA and enhance its translation into therapeutic proteins.
- Antisense oligonucleotides (ASOs): ASOs are being developed to treat a variety of genetic diseases, including spinal muscular atrophy and Huntington's disease.
Moving Forward
The development and manufacture of oligonucleotides is a complex process that requires careful attention to design, synthesis, purification, and quality control. Advances in synthesis techniques, delivery systems, and regulatory frameworks are driving the expansion of oligonucleotide applications, particularly in the therapeutic and biotechnology sectors. However, challenges such as ensuring specificity, stability, and regulatory compliance remain critical.
The EMA’s new guideline on the development and manufacture of oligonucleotides provides a comprehensive framework for the development and production of oligonucleotides, emphasizing adherence to best practices and regulatory standards to meet the growing demands of medical and biotechnological applications.
By following best practices and leveraging emerging technologies, the industry can continue to develop high-quality oligonucleotides to meet the growing demand for innovative genetic solutions.
References
- Thomas Roberts et al., Nature Reviews Drug Discovery 2020; (https://doi.org/10.1038/s41573-020-0075-7)
- R Obexer et al., Science 2024 (DOI: 10.1126/science.adl4015)
- Christine Roden and Amy Gladfelter, Nature Reviews Molecular Cell Biology 2021; (https://doi.org/10.1038/s41580-020-0264-6)
- Martin Frandsen et al., Angewandte 2023; (https://doi.org/10.1002/anie.202303170)
- Wout Bittremieux et al., Mass Spectrometry Reviews 2017; (https://doi.org/10.1002/mas.21544)
- Aleksei Yantsevich et al., SLAS Technology 2019; (https://doi.org/10.1177/2472630319850534)
- Patrik Andersson, Antisense RNA Design, Delivery, and Analysis 2022; (https://www.ncbi.nlm.nih.gov/books/NBK584239/)
- Alexander Sandahl et al., Nature Communications 2021; (https://doi.org/10.1038/s41467-021-22945-z)
- Robert Minkner et al., Electrophoresis 2022 (doi: 10.1002/elps.202200079)
- Stefan Bugovsky et al., Rapid Communications in Mass Spectrometry 2016 (https://doi.org/10.1002/rcm.7471)
- Olga Gonzalez-Gonzalez et al., Pharmaceutics 2022 (10.3390/pharmaceutics14112324)
- Michael Pennington et al., Medicine in Drug Discovery 2021 (https://doi.org/10.1016/j.medidd.2020.100071)
- Thomas Rupp and Hagen Cramer, RNA Therapeutics 2022 (https://doi.org/10.1016/B978-0-12-821595-1.00012-9)
- Alex Hoose et al., Nature Reviews Chemistry 2023 (https://doi.org/10.1038/s41570-022-00456-9)
- Thomas Roberts et al., Nature Reviews Drug Discovery 2020 (https://doi.org/10.1038/s41573-020-0075-7)
- Peter Kirkpatrick, Nature Biopharma Dealmakers 2023 (https://doi.org/10.1038/d43747-023-00104-1)
About The Author:
Jack (Jie) Huang, MD, Ph.D., is the chief scientist and founder/CEO at CSTEAM Biotechnology in Ohio, USA. He is also recognized as a medical science writer, an inventor, and a STEM educator. Huang completed his MD/Ph.D. at Shiga University of Medical Science (SUMS) in Japan in 2000. He then pursued postdoctoral training in immunology at the University of Tokyo, followed by a role as research assistant professor at Tohoku University. In the U.S., he served as a postdoctoral fellow at Oregon Health & Science University and as an oncologist and senior research scientist at Ohio State University Nationwide Children’s Hospital, focusing on oncology research until 2018, when he launched his company specializing in biological models and biochips. Additionally, Huang is vice president of the American Botanical Drug Association and director of the Stem Cell Engineering and Technology Research Center at the Industry Technology Research Institute of Chongqing University. His research interests, which span genetic engineering biological models, gene-edited stem cells, immune cell drugs, and biochip technology, have led to numerous patents.