CRISPR-Cas Genome Editing For Rejuvenation Of Aging Stem Cells
By Jie Huang, MD/Ph.D., CSTEAM-Biotech
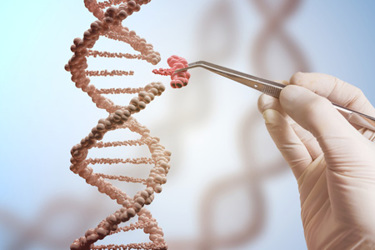
CRISPR-Cas genome editing technology offers groundbreaking potential for restoring the vitality of aged stem cells, a major advance that promises to revolutionize regenerative medicine and aging research. As stem cells age, their regenerative capacity declines due to accumulated genetic and epigenetic changes. Using CRISPR-Cas, scientists can directly edit these changes or modulate aging-related pathways, potentially restoring or enhancing the function of these cells. This technology offers promise for treating age-related diseases by improving the health and function of stem cells, thereby opening new therapeutic avenues and extending the quality of life of older adults.
Mechanisms And Methods
Targeting aging-related genes through CRISPR technology provides a promising strategy to combat cellular aging and extend the functional lifespan of stem cells. CRISPR can be used to specifically delete or modify genes that contribute to senescence, such as those involved in the p16INK4a-pRB and p53-p21 pathways, which are key regulators of the cell cycle and cellular senescence.1,2 By editing these key aging-related genes, the occurrence of stem cell aging can be delayed, and their regenerative capacity and vitality can be enhanced. This approach not only has the potential to significantly extend the functional lifespan of stem cells but also offers broad therapeutic implications for age-related diseases and conditions, promoting healthier aging and increasing overall lifespan.2
Epigenetic rejuvenation is a cutting-edge approach to address the decline in stem cell function due to aging, which is often marked by changes in epigenetic marks that affect gene expression without altering the DNA sequence.3,4 Over time, these epigenetic alterations lead to a decline in cell viability and regenerative capacity. Utilizing CRISPR-based tools, such as dCas9 (dead Cas9) fused to epigenetic modifiers, provides a sophisticated approach to reversing these changes.3 dCas9 does not cut DNA but can be targeted to specific gene loci, where it delivers epigenetic enzymes that alter the state of chromatin. By strategically targeting and modifying epigenetic marks, such as DNA methylation or histone acetylation, silent genes that are critical for cell viability and stem cell function can be reactivated.4 The technology effectively rejuvenates stem cell populations, restoring their ability to function properly and enhancing their therapeutic potential.4 The implications of this technology are profound, as it has the potential to not only extend the lifespan of stem cells but also improve the efficacy of stem cell-based therapies for age-related diseases. This approach provides a powerful tool for regenerative medicine that aims to restore youthful characteristics at the cellular level and promote healthier aging outcomes.3,4
Figure 1: CRISPR-Cas-based Genome Editing. The diagram depicts the CRISPR-Cas9 genome editing process. Cas9, guided by a specific guide RNA, binds to a matching sequence on genomic DNA, inducing a double-strand break. This directed cleavage allows modifications to be introduced via a repair mechanism that utilizes the donor DNA as a template. The process results in precise genetic alterations. The diagram highlights the application of this technology in various biological systems, including humans, mice, and isolated cells, demonstrating the versatility and precision of CRISPR-Cas9 in editing genetic information in different organisms for research or therapeutic purposes.
Telomere extension using CRISPR technology offers a promising approach to combat cellular aging. Telomeres are protective caps at the ends of chromosomes that naturally shorten as cells divide and age, leading to cellular aging and loss of function. By targeting and editing the TERT gene, which encodes the telomerase reverse transcriptase, CRISPR can reactivate telomerase expression in senescent cells. This reactivation lengthens telomeres, thereby increasing the lifespan and functional capacity of these cells.5 These advances have the potential not only to extend cellular health and lifespan but also to improve treatments for age-related diseases, bringing major breakthroughs to regenerative medicine and anti-aging treatments.
Challenges And Considerations
Precision in genome editing is critical, especially when using technologies such as CRISPR-Cas9, as there is a risk of off-target effects when edits occur at unintended sites in the genome. Such off-target edits can lead to serious unintended consequences, including genomic instability and carcinogenesis.1 These unintentional changes may activate oncogenes or inactivate tumor suppressor genes, increasing the risk of cancer. To reduce these risks, it is essential to enhance the specificity of CRISPR components and employ rigorous screening methods to detect and minimize off-target activity.2 Advances in CRISPR technology and computational tools continue to increase precision, helping to reduce potential risks and ensure safer treatment outcomes.
Efficient delivery of CRISPR components to stem cells, especially in vivo, presents significant challenges, and addressing these challenges is critical to the success of CRISPR-based therapies.7 Achieving high editing efficiency while ensuring safe and targeted delivery of these components to the desired cells without affecting other cell types is a complex task. Several approaches are currently being explored to improve delivery efficiency. These approaches include viral vectors, which are highly efficient at transducing cells but have limitations such as potential immunogenicity and insertional mutagenesis. Alternatively, non-viral approaches such as lipid nanoparticles, electroporation, and microinjection are being optimized to reduce the risks associated with viral vectors and improve targeting precision.8 The efficiency of CRISPR editing itself is critical. It is not enough to simply deliver the components; they must be efficient in inducing the desired genetic changes. This requires optimizing the specificity of the guide RNA, using high-fidelity Cas enzymes to reduce off-target effects, and enhancing homology-directed repair mechanisms, which are often inefficient in adult stem cells.9 In addition, the development of controlled-release systems that enable sustained release of CRISPR components, as well as the use of tissue-specific promoters to restrict expression of these components in target cells, are strategies being developed to increase the precision and safety of CRISPR-based therapies. Addressing these challenges will be critical to translating the promise of CRISPR-mediated stem cell therapies into practical, reliable medical treatments.
Stem cell regeneration through manipulation of fundamental biological processes raises significant ethical and regulatory issues. These issues require rigorous oversight, especially when the therapies are used in humans. Ethical debates focus on the impact of genetic manipulation, potential long-term effects, and the morality of altering human biology at the cellular level.3,4 Regulators must ensure that these therapies are safe, effective, and ethical and adopt comprehensive guidelines to govern clinical trials and eventual clinical use to protect the well-being of patients.
Applications In Regenerative Medicine, Aging, And Age-Related Disease
The therapeutic potential of regenerative stem cells is enormous, particularly in treating age-related diseases where natural tissue regeneration is impaired. These stem cells can be used in cell therapies to repair or replace damaged tissue in diseases such as osteoporosis, which causes a loss of bone density; neurodegenerative diseases such as Alzheimer's and Parkinson's, where neuronal cells are progressively lost; and heart disease, where damage to cardiac tissue often requires regenerative intervention to restore function.10 By restoring or replacing dysfunctional cells, stem cell therapies can reverse disease symptoms, slow disease progression, and improve quality of life. In addition, these therapies are being explored for their ability to treat age-related macular degeneration and other forms of chronic tissue inflammation. The integration of advanced gene editing technologies such as CRISPR further improves the effectiveness and specificity of these treatments, opening new avenues for personalized medicine for the aging population.1
Enhanced stem cells, driven by advances in gene editing and cell culture technologies, offer transformative potential for research and drug discovery, particularly in the study of aging and age-related diseases.10 These cells provide a highly relevant model system that can mimic the complex biological processes associated with aging more accurately than traditional models. This relevance is critical for uncovering the molecular and cellular mechanisms behind diseases that primarily affect older adults, such as Alzheimer's disease, Parkinson's disease, osteoarthritis, and cardiovascular disease. Using stem cells, researchers can create disease-specific or patient-specific cell lines.2 For example, cells from patients with genetic neurological diseases can be reprogrammed into pluripotent stem cells and then differentiated into neuronal cells to study disease progression and pathology. This approach allows for the identification of new drug targets and screening of potential treatments in a controlled but biologically relevant environment.3 In addition, these enhanced stem cells can be used in a high-throughput screening setting. Various cell types derived from these stem cells, including cardiomyocytes, neurons, or osteoblasts, can be efficiently tested to discover drugs that may reverse or slow the aging process.4 This approach not only accelerates the pace of drug discovery but also improves its accuracy by providing early insights into the efficacy and safety of potential treatments. Additionally, the use of stem cells in research advances the study of the aging process itself, providing valuable data that can inform preventive strategies and overall treatments.4 As our understanding of how stem cells change with age and how these changes affect their regenerative capacity continues to improve, we can develop better interventions to target these processes, potentially leading to breakthrough preventive measures or therapies that extend healthy lifespan and address the root causes of age-related diseases.
Overall, CRISPR-Cas genome editing is a powerful tool for addressing aging at the cellular level, providing a promising strategy for extending cellular health and lifespan. The technology enables precise modifications to DNA that can correct age-related genetic defects, enhance cellular function, and potentially delay the onset of aging symptoms. By manipulating key genes involved in the aging process, CRISPR-Cas has the potential to revolutionize our approach to promoting healthy aging and achieving longevity, changing treatment strategies and improving quality of life.
References
- Campisi, J., & d'Adda di Fagagna, F. (2007). Cellular senescence: when bad things happen to good cells. Nature Reviews Molecular Cell Biology, 8(9), 729-740.
- Jinek, M., Chylinski, K., Fonfara, I., Hauer, M., Doudna, J. A., & Charpentier, E. (2012). A programmable dual-RNA–guided DNA endonuclease in adaptive bacterial immunity. Science, 337(6096), 816-821.
- Horvath, S., & Raj, K. (2018). DNA methylation-based biomarkers and the epigenetic clock theory of ageing. Nature Reviews Genetics, 19(6), 371-384.
- Izpisua Belmonte, J. C., & Ocampo, A. (2021). Cellular rejuvenation therapy and the dawn of regeneromics. Nature, 601(7893), 195-203.
- Liu, L., Bailey, S. M., Okuka, M., Munoz, P., Li, C., Zhou, L., Wu, C., Czerwiec, E., Sandler, L., Seyfang, A., Blasco, M. A., & Keefe, D. L. (2007). Telomere lengthening early in development. Nature Cell Biology, 9(12), 1436-1441.
- Lu, Y., Xue, J., Deng, T., Zhou, X., Yu, K., Deng, L., Huang, M., Yi, X., Liang, M., Wang, Y., & Dai, Z. (2020). Safety and feasibility of CRISPR-edited T cells in patients with refractory non-small-cell lung cancer. Nature Medicine, 26(5), 732-740.
- Senís, E., Fatouros, C., Große, S., Wiedtke, E., Niopek, D., Mueller, A. K., Börner, K., & Grimm, D. (2014). CRISPR/Cas9-mediated genome engineering: An adeno-associated viral (AAV) vector toolbox. Biotechnology Journal, 13(11), 1700416.
- Bak, R. O., Dever, D. P., & Porteus, M. H. (2018). CRISPR/Cas9 genome editing in human hematopoietic stem cells. Nature Protocol, 13(2), 358-376.
- Ocampo, A., Reddy, P., Martinez-Redondo, P., Platero-Luengo, A., Hatanaka, F., Hishida, T., ... & Izpisua Belmonte, J. C. (2016). In vivo amelioration of age-associated hallmarks by partial reprogramming. Cell, 167(7), 1719-1733.e12.
- Sarkar, T. J., Quarta, M., Mukherjee, S., Colville, A., Paine, P., Doan, L., ... & Rando, T. A. (2020). Transient non-integrative expression of nuclear reprogramming factors promotes multifaceted amelioration of aging in human cells. Nature Communications, 11(1), 1545.
About The Author:
Jack (Jie) Huang, MD, Ph.D., is the chief scientist and founder/CEO at CSTEAM Biotechnology in Ohio, USA. He is also recognized as a medical science writer, an inventor, and a STEM educator. Huang completed his MD/Ph.D. at Shiga University of Medical Science (SUMS) in Japan in 2000. He then pursued postdoctoral training in immunology at the University of Tokyo, followed by a role as research assistant professor at Tohoku University. In the U.S., he served as a postdoctoral fellow at Oregon Health & Science University and as an oncologist and senior research scientist at Ohio State University Nationwide Children’s Hospital, focusing on oncology research until 2018, when he launched his company specializing in biological models and biochips. Additionally, Huang is vice president of the American Botanical Drug Association and director of the Stem Cell Engineering and Technology Research Center at the Industry Technology Research Institute of Chongqing University. His research interests, which span genetic engineering biological models, gene-edited stem cells, immune cell drugs, and biochip technology, have led to numerous patents.