An Independent Expert's Guide To Picking Nanoparticle Manufacturing Equipment
By Alexander K. Aust, M.S., MBA, Aust Business Solutions
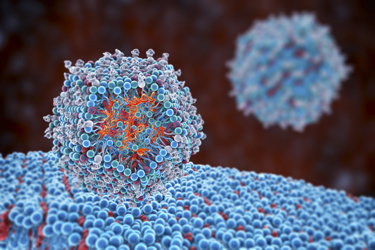
Oligonucleotide, small molecule, and bioactive lipid therapeutics are beginning to play a larger role in the treatment of many infectious diseases and cancers. However, these therapeutics are highly unstable, are degraded when administered, and are often inhibited from crossing certain membrane barriers due to their physical size and negative charge.1
Nanoparticle manufacturing, which overcomes these barriers, has become an increasingly popular apparatus for drug delivery systems (DDS). There are several methods for screening lipids or polymers for use in DDS formulation (i.e., mixing methods), such as emulsification, micromixing, extrusion/homogenization, solvent injection, and microfluidic methods. Drug product characterization is key. There are several methods for characterization, such as dynamic light scattering (DLS), zeta potential, HPLC, and more.
These methods look for particle size, purity, composition, encapsulation efficiency (EE), charge, and polydispersity.2 Ensuring that the chosen manufacturing equipment can uphold these specific characteristics is critical in producing the perfect nanoparticle.
Clinical Or Commercial Batches? Scale Determines What Equipment You Need
Choosing the correct nanoparticle manufacturing equipment is dependent on the scale of nanoparticle manufacturing required. Some equipment is scaled to research and development (R&D), while others are designed for large-scale use in accordance with good manufacturing practice (GMP).
The various methods used for formulating pharmaceutical-grade nanoparticles are given in the table below. The table provides the commercially available options for procurement. The equipment characteristics you choose, such as max flow rate, min/max sample (i.e., batch) volume, and EE, ultimately have a large impact on what nanoparticles can be produced. This is especially true of the various lipid screening requirements for lipid nanoparticle (LNP) formulation development in the preclinical phase. Interestingly, manufacturing large batches of LNP drug products has been historically “easier” to perform than manufacturing very small batches.
Until recently, this meant that drug sponsors would have to switch between technology types between the preclinical, clinical, and commercial phases. Over the past two years, there has been a race to the bottom for nanoparticle equipment innovation to determine which companies can provide solutions at the very smallest scales required in early development and large batches in the later clinical/commercial stages.
Nanoparticle Manufacturing Equipment |
||||||
Company |
Model |
Technology |
R&D/ cGMP |
Max Flow Rate |
Min/Max Volume |
EE |
Avanti |
Extrusion |
R&D |
* |
.025 mL / 1 mL |
* |
|
CDMO |
In-house1 |
Solvent Injection |
R&D/GMP |
As Needed |
As Needed |
* |
CDMO |
In-house1 |
Extrusion |
R&D/GMP |
As Needed |
As Needed |
* |
DIANT |
Solvent Injection |
R&D |
400 mL/min |
20 mL / * |
* |
|
DIANT |
Solvent Injection |
GMP |
2 L/min |
* / * |
* |
|
DIANT |
Solvent Injection |
GMP |
20 L/min |
* / * |
* |
|
Evonik |
Extrusion |
R&D |
* |
1 mL / > 100 L |
>90% |
|
FDX Fluid Dynamix |
Solvent Injection |
R&D/GMP |
>1 L/min |
0.75 mL / * |
>95% |
|
GEA |
Homogenization |
R&D/GMP |
9 L/hr |
* / * |
* |
|
GEA |
Homogenization |
R&D/GMP |
>1,500 L/hr |
* / * |
* |
|
GEA |
Homogenization |
GMP |
10,000 L/hr |
* / * |
* |
|
GEA |
Homogenization |
GMP |
1,100 L/hr |
* / * |
* |
|
Helix Biotech |
Solvent Injection |
R&D |
200 mL/min |
0.1 mL / 50 mL |
* |
|
Inside Therapeutics |
Microfluidic |
R&D |
30 mL/min |
0.025 mL / 0.25 mL |
>90% |
|
Inside Therapeutics |
Microfluidic |
R&D |
* |
* / 1 L |
>90% |
|
Knauer |
Solvent Injection |
R&D/GMP |
70 mL/min |
1 mL / 10 L |
>90% |
|
Knauer |
Solvent Injection |
cGMP |
60 L/min |
10 L / 100 L |
>90% |
|
Knauer |
Solvent Injection |
cGMP |
120 L/hr |
10 L / >1,000 L |
>90% |
|
Leon Nanodrugs |
Solvent Injection |
R&D |
* |
* / * |
* |
|
Leon Nanodrugs |
Solvent Injection |
cGMP |
* |
* / * |
* |
|
Leon Nanodrugs |
Solvent Injection |
cGMP |
* |
* / * |
* |
|
Microfluidics |
Homogenization |
R&D |
600 mL/min |
30 mL / * |
* |
|
Microfluidics |
Homogenization |
R&D |
90 mL/min |
14 mL / * |
* |
|
Microfluidics |
Homogenization |
GMP |
120 mL/min |
50 mL / * |
* |
|
Microfluidics |
Homogenization |
GMP |
450 mL/min |
120 mL / 100 L |
* |
|
Microfluidics |
Homogenization |
GMP |
1.2 L/min |
1.5 L / * |
* |
|
Microfluidics |
Homogenization |
GMP |
7.56 L/min |
5.0 L / * |
* |
|
Microfluidics |
Homogenization |
GMP |
15.12 L/min |
5.0 L / * |
* |
|
Micropore |
Micromixing |
R&D |
150 mL/min |
1 mL / 10 L |
97% |
|
Micropore |
Micromixing |
GMP |
200 L/hr |
5 mL / >100 L |
98% |
|
Micropore |
Micromixing |
GMP |
1,500 L/hr |
30 mL / >1,000 L |
* |
|
Micropore |
Micromixing |
R&D |
200 mL/min |
0.2 mL / 250 mL |
* |
|
Particle Works |
Microfluidic |
R&D |
30 mL/min |
0.1 mL / 2 mL |
98% |
|
Particle Works |
Microfluidic |
R&D |
30 mL/min |
0.25 / 10 mL |
>90% |
|
PNI |
NxGen™ Mixing |
R&D |
N/A |
0.025 mL / 0.25 mL |
>90% |
|
PNI |
NxGen™ Mixing |
R&D |
200 mL/min |
1.0 / 60 mL |
>90% |
|
PNI |
NxGen™ Mixing |
R&D |
115 mL/min |
10 mL / 10 L |
>90% |
|
PNI |
NxGen™ Mixing |
GMP |
200 mL/min2 |
10 mL / * |
>90% |
|
PNI |
NxGen™ Mixing |
GMP |
48 L/hr |
0.5 / 400 L |
>90% |
|
PNI |
Modular Commercial Formulation Skid3 |
NxGen™ Mixing |
GMP |
48 L/hr |
* / 400 L4 |
>90% |
PreciGenome |
Microfluidic |
R&D |
3 mL/min |
0.1 mL / 2 mL |
>90% |
|
PreciGenome |
Microfluidic |
R&D |
3 mL/min |
1 mL / 12 mL |
>90% |
|
PreciGenome |
Microfluidic |
R&D |
12 mL/min |
2 mL / 200 mL |
>90% |
|
PreciGenome |
Microfluidic |
GMP |
>1 L/hr |
10 mL / >1 L |
>90% |
|
Unchained Labs |
Microfluidic |
R&D |
30 mL/min |
0.25 mL / 1 L |
≥85% |
|
* Unknown due to mixed reports or a lack of information/data published. 1 Nonproprietary equipment created by the manufacturing site to facilitate technology transfers into their facilities. 2 Upgrade to the GMP unit available that allows for max flow rate = 1.6 L/min. 3 Commercial launches scheduled for mid-July 2023. Information provided by PNI representative. 4 Maximum amount per mixing module. |
The characteristic EE is of specific interest to drug sponsors with expensive API. EE refers to the percentage of nanoparticles that have encapsulated the target payload.3 Take note of the many factors that influence EE from either the nanoparticle or therapeutic, such as hydrophilicity, surface area, aqueous volume, preparation method, and many others.3 This also means that an encapsulation rate of 95% does not equal 95% encapsulation of all API types within an LNP.
However, this does mean that this specific particle size encapsulates 95% of the API within the nanoparticles. Ideally, EE should be at or above 95% due to the high costs associated with nucleic acids (mRNA, DNA, etc.) and the desire to process more samples with less volume/loss. High EE corresponds to higher drug efficacy. Note that this is pulling from API+LNP data; there are many different EEs associated with different API+LNP combinations and thus it is equipment and formulation specific.
In addition, the flow rates of different equipment play a pivotal role in the physicochemical characteristics of nanoparticle formation.4 An increased flow rate decreases particle size, which is ideal for producing particles that are 100 nm or smaller. In addition to manipulating particle size, the nanoparticle manufacturing rate can also be manipulated with varying flow rates.4 Minimum and maximum sample volume production should also be considered when looking at equipment. Using equipment with higher sample volume production can decrease manufacturing time and increase sample preparation.
Micromixing, Solvent Injection, And Other Preparation Methods
It is also important to choose the appropriate method of nanoparticle screening dependent on the API, lipid, and scale of manufacturing interest. The newest screening method is microfluidics. This method is known for precisely controlling LNP size, broadband LNP formulation optimization, reproducibility, and LNP processes that are continuous.5 Microfluidic methods can be split into two general approaches: traditional microfluidics and micromixing. Traditional microfluidics involves the mixing of two liquids and a solid to synthesize nanoparticles via laminar flow.5 The devices that utilize traditional microfluidics must be carefully designed to limit nanoparticle disposition via channel blockage.
In contrast, micromixers refer to the synthesis of organic nanoparticles compared to traditional liquid-liquid mixing.6 There are two main designs of micromixers: hydrodynamic flow focusing and staggered herringbone. Hydrodynamic flow focusing (HFF) mixing uses the laminar flow design. A stream of solvent dissolved nanoparticle precursors flows parallel to a stream of antisolvent.6 These parallel streams allow the mixture of the solutions by diffusion.
On the other hand, staggered herringbone micromixing uses chaotic advection caused by herringbone structures dependent on six geometric parameters.6 The chaotic advection from the system allows for smaller mixing times at lower fluid rates to produce nanoparticles more quickly while limiting dilution rates.
Although microfluidics is a great tool for nanoparticle formation, until recently it has only been suited for small-scale production. The solvent injection method is generally used to keep up with high volume demands for clinical and commercial launches.
Solvent injection works by dissolving the nanoparticles and API in a water-miscible solvent along with an aqueous phase via the addition of an emulsifier.7 The organic phase is injected into the aqueous phase, where droplet reduction occurs. The reduction forms the nanoparticles and the emulsifier stabilizes them.7 The advantages of using solvent injection include the mechanism’s simplicity, reproducibility, and scalability. This technology also lends itself to a continuous manufacturing method, as opposed to a batch-style method. In the former method, you just “set it, and forget it.”
Homogenization and extrusion are other ways to prepare nanoparticles on a large scale. Homogenization uses extreme pressure conditions to reduce particles to a uniform desired size.7 Coarse lipid particles are synthesized and pushed through a homogenizer with small gaps. The advantages of homogenization include scalability, quickness, and low cost. Extrusion is a method that forces large particles through nanopore channels to form the final LNP product.8
About the Author:
Alexander Aust, M.S., MBA, is the cofounder and owner of Aust Business Solutions, a pharmaceutical consultancy based in Maryville, TN, where he focuses on strategic planning and partnerships and technical support. The firm provides CMC and project management services, assists with regulatory submissions, and helps to implement new technology. Aust serves as a senior project manager for the Parenteral Drug Association. He earned his MBA from Ball State University and an M.S. degree from Purdue University in molecular and cellular biology.
References:
- http://dx.doi.org/10.2174/2211738507666190925112942
- https://www.mdpi.com/1999-4923/12/11/1095
- https://www.frontiersin.org/articles/10.3389/fnano.2021.719710/full
- https://www.ncbi.nlm.nih.gov/pmc/articles/PMC7697682/
- https://www.sciencedirect.com/science/article/pii/S0168365922000918
- https://onlinelibrary.wiley.com/doi/10.1002/smll.202106580
- https://www.ncbi.nlm.nih.gov/pmc/articles/PMC7587569/
- https://www-leland.stanford.edu/group/Zarelab/publinks/985.pdf
For more information, see also https://www.austbs.com/post/nanoparticle-manufacturing-equipment-updated